On Sunday, August 12, 2018, an extraordinary mission took place: the launch of the Parker Solar Probe. This probe embarked on a journey into interplanetary space, but its destination is not another planet. No, it’s not aiming for a circular orbit to observe our star from afar. Instead, the Parker Solar Probe is set to approach the Sun as close as possible to measure and study its corona. This incredible mission was undertaken by NASA and is expected to last for nearly seven years, thanks to equipment specially prepared for the probe.
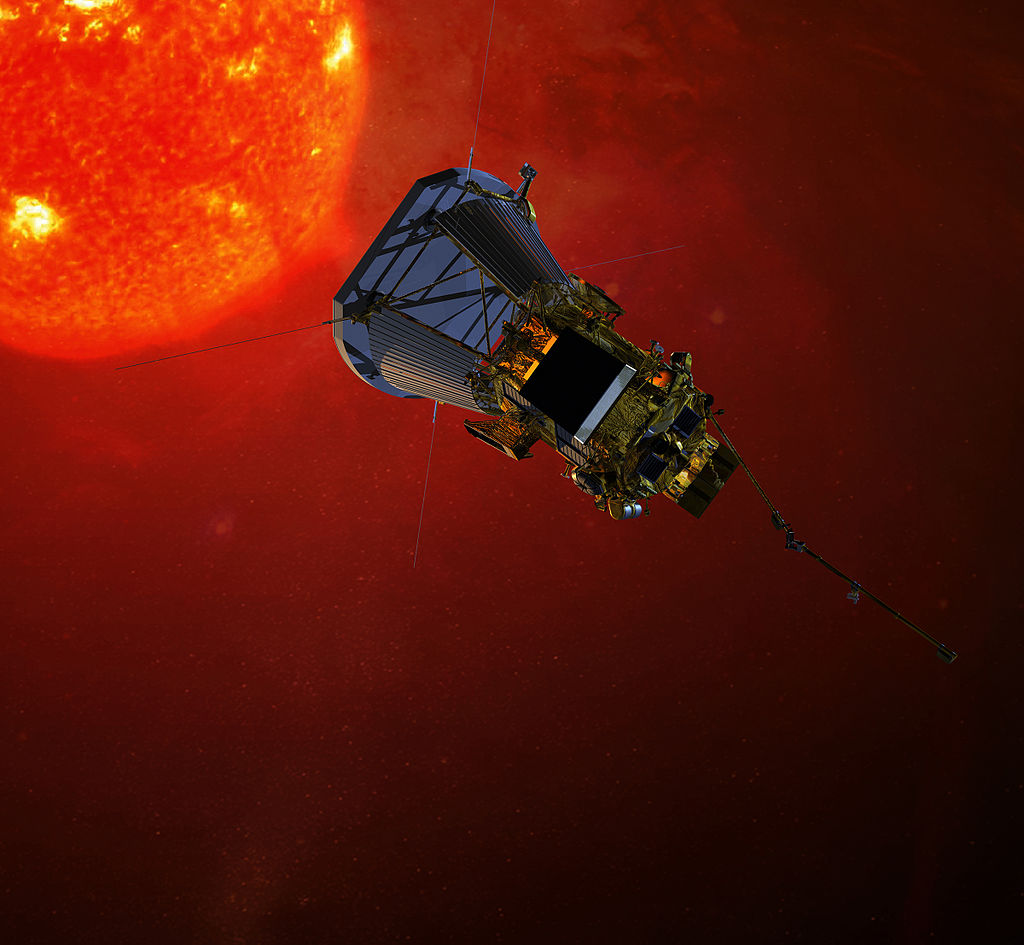
Histoire
The PSP (Parker Solar Probe) mission originated from the American Solar Probe project created in 2005. At that time, New Horizons, the probe that explored Pluto and will soon fly by another object in the Kuiper Belt, was in full production. With New Horizons, there would be only one area of our solar system left unexplored: the region near the Sun. NASA launched a feasibility study for such a mission in 2004-2005. The results were very promising! The mission was planned to send a probe to Jupiter to take advantage of its gravity to deflect it onto a polar orbit with a perihelion of less than four solar radii. However, the problem was that its aphelion would be at Jupiter’s orbit, and the orbital period would be so long that it would only allow for two solar flybys. Due to this distant aphelion, the probe would have needed to use radioisotope thermoelectric generators (RTGs) to provide power. This proposed mission was technically feasible but would have cost at least $1.1 billion, a price tag deemed too high for such a probe.
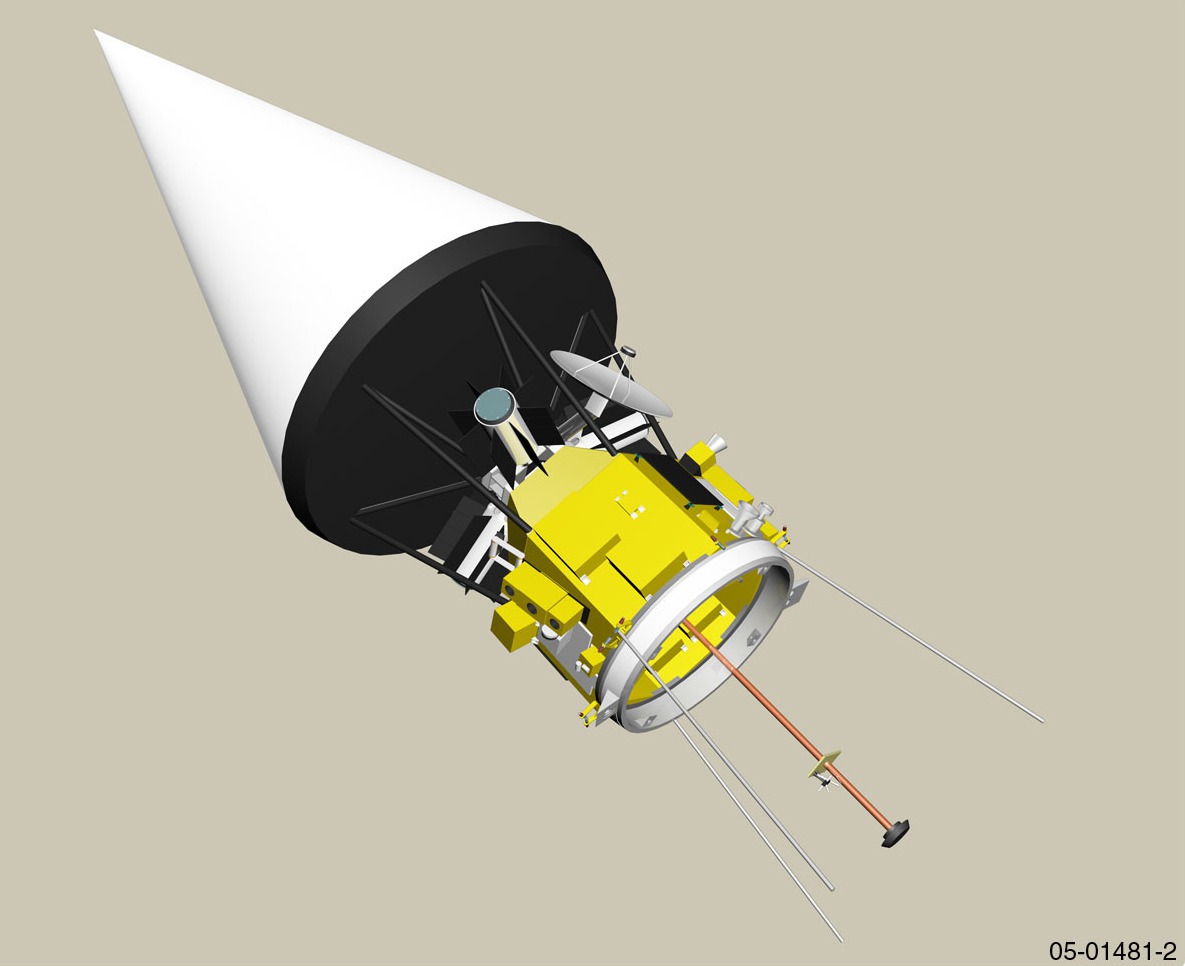
A few years later, NASA initiated a new study to carry out a similar mission but with two new constraints: generating power without RTGs and keeping the total cost below $750 million. In 2008, the Solar Probe Plus project was born, and its characteristics were redefined. To avoid the use of RTGs, the decision was made to utilize numerous gravitational assists from Venus instead of a single one from Jupiter. In addition to bringing the aphelion of the orbit closer, these assists also extended the duration of study time around the Sun. While Solar Probe had 160 hours of study time, Solar Probe Plus increased this to 2,100 hours, thanks to the significantly higher number of flybys (24 instead of 2). Another advantage was a slower velocity during the solar flyby, providing even more observation time: 195 km/s compared to the previous maximum of 308 km/s. The only drawbacks were a slightly greater distance from the Sun (minimum of 10 solar radii), offset by reduced thermal constraints, and the loss of the ability to study the solar poles. However, the polar study aspect would be undertaken by the ESA’s Solar Orbiter, scheduled for launch in 2020. With the overall plan in place, the focus shifted to fabrication and, most importantly, solving the major temperature challenge. Indeed, Solar Probe Plus would face temperatures exceeding 1,400K (1,127°C) due to a solar wind 500 times more energetic than that experienced in Earth’s orbit.
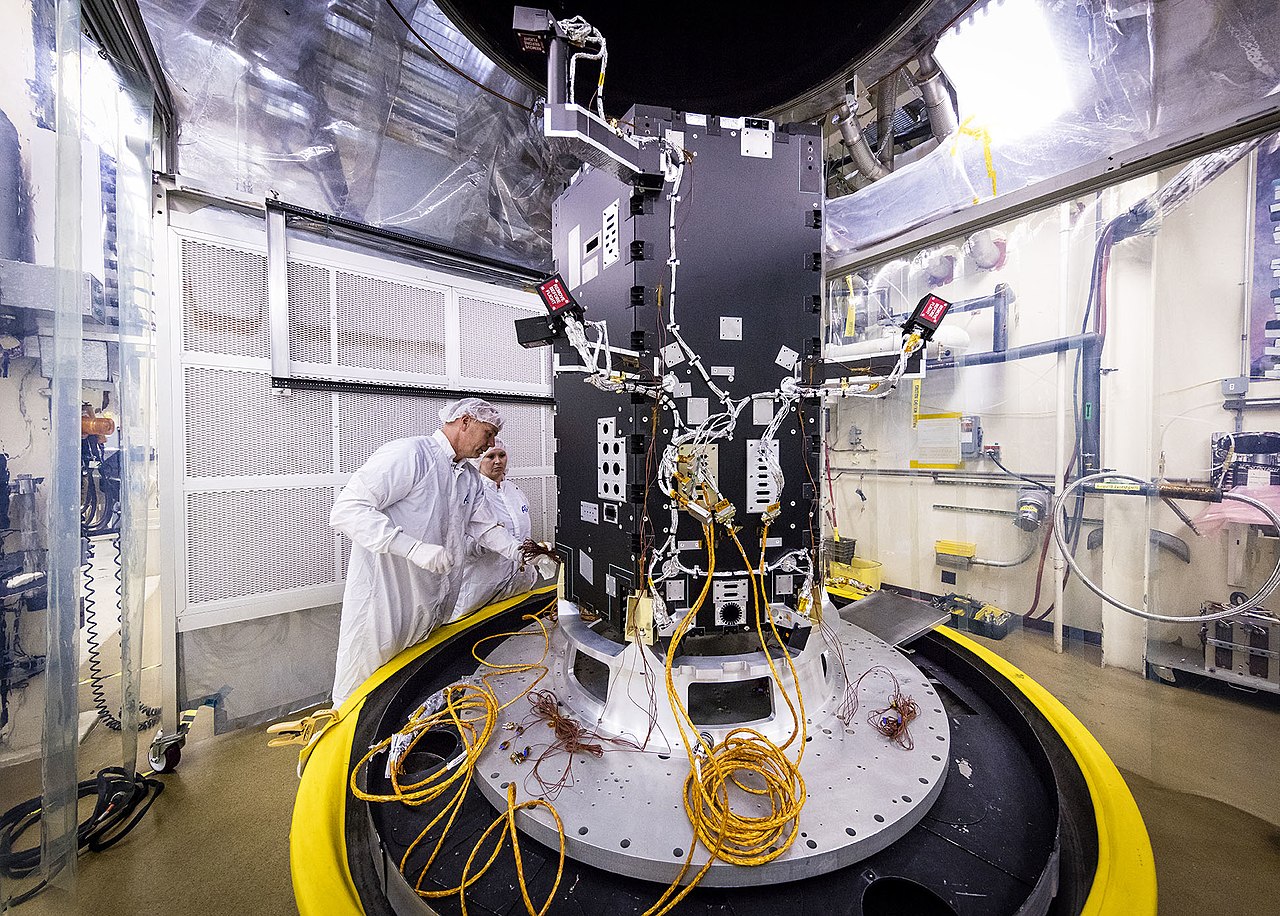
Thus, in 2008, the budget needed for the mission was allocated, and the project was assigned to NASA’s Goddard Space Flight Center, which was already conducting studies on the influence of our star on our planet with its Living With a Star project. NASA entrusted the Johns Hopkins University Applied Physics Laboratory with the design and development of the satellite. By the end of the following year, the same laboratory completed a preliminary study on the new technologies that needed to be invented: thermal shield, solar panels, cooling system. Construction of the probe began in the following years, and the launch date was scheduled for August 2018, an ideal date to perform all the desired gravitational assists. A backup launch window was planned for May of the following year, but it required an additional Venus flyby. In May 2017, NASA decided to rename the probe Parker Solar Probe in honor of astrophysicist Eugene Parker. Sixty years ago, Parker hypothesized that all stars continuously emit a stream of energetic particles. This stream, verified and measured in the following decades by space missions, was named the “solar wind.” Interestingly, Parker Solar Probe is NASA’s first probe whose name comes from a person still alive at the time of launch. In the end, the development cost of the mission was estimated at $1.05 billion, and the preliminary studies, launch, and operational management costs at $530 million: a reminder that final budgets often exceed initial budgets.
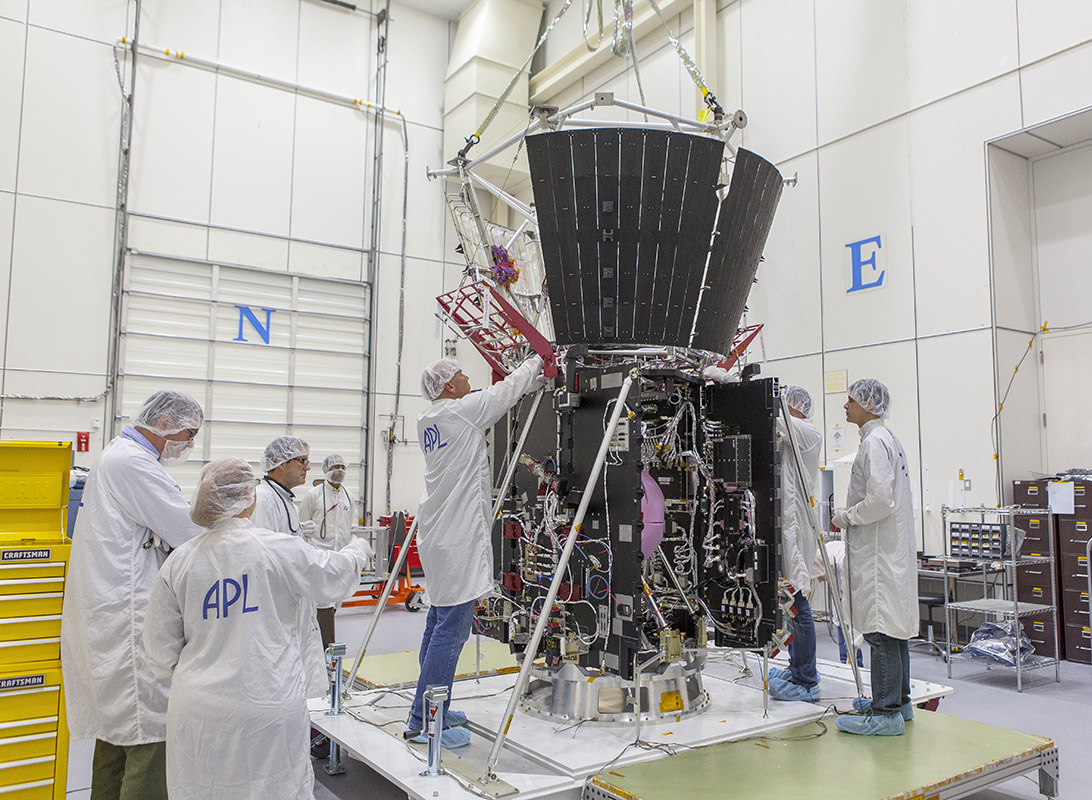
Scientific Instruments
The goal of PSP is to study the solar corona. This layer of the Sun extends over several million kilometers, reaches a temperature of one million Kelvin (compared to 5500K for the solar surface), and is responsible for solar winds: streams of very energetic particles that bombard all bodies in the solar system. Over the past decades, our understanding of this region has continued to grow, but the phenomena of heating and acceleration of solar winds remain a mystery. Parker Solar Probe will thus have four objectives:
- Determine the structure and evolution of the magnetic fields responsible for both slow and fast solar wind particles,
- Trace the energy flows that heat the solar corona and accelerate solar wind particles,
- Determine the processes behind the acceleration and transport of energetic particles,
- Study the phenomenon of “dusty plasma” (see image below) near the Sun and its influence on solar wind and the formation of energetic particles.
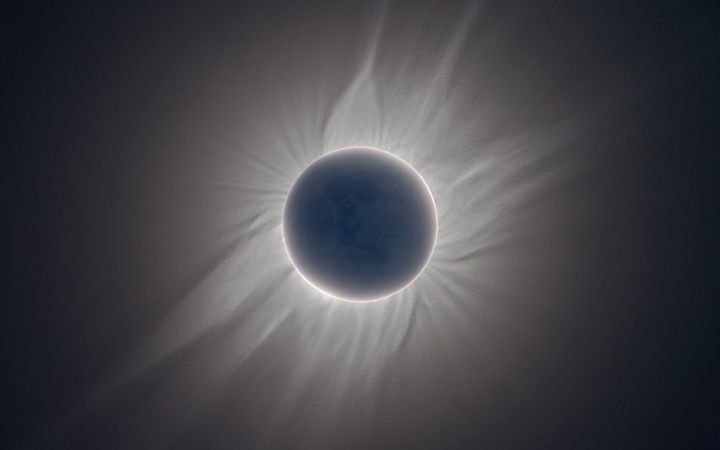
To achieve all of this, Parker Solar Probe is equipped with four scientific instruments, which are listed below along with their explanations:
WISPR : Le coronographe Wide-field Imager for Solar Probe (Imageur grand champ pour sonde solaire) est une caméra grand angle qui fournira des images en trois dimensions de la couronne solaire. Si on considère que la direction du Soleil est 0°, WISPR couvrira la zone comprise entre 13,5° et 108°. Ce coronographe est caché dans l’ombre du bouclier thermique de Parker Solar Probe (dont nous reparlerons plus tard). Etant donné qu’au périhélie, le Soleil aura une taille apparente de 12°, WISPR a une marge de 7,5° d’occultation (13,5 – 12 / 2) qui lui évite d’être abîmé en cas d’anomalies dans le pointage de la sonde. Cet instrument est placé de telle manière qu’il image dans le sens de déplacement de Parker Solar Probe. Ainsi, les phénomènes qui seront détectés in situ par les autres instruments pourront être identifiées grâce à WISPR. Ce dernier est composé de deux télescopes : l’un couvrant l’angle interne (de 13,5° jusqu’à 53°) et l’autre couvrant l’angle externe (de 53° à 108°). Le capteur CCD de ce coronographe mesure 2048 pixels par 1920 soit des images de presque 4 mégapixels. Au final, la résolution angulaire (angle au quel correspond un pixel sur l’image) est de 17 secondes d’arc quand la sonde est au plus proche du Soleil. WISPR a été développé et fabriqué par le Naval Research Laboratory en Caifornie sous la direction du professeur Russell Howard.
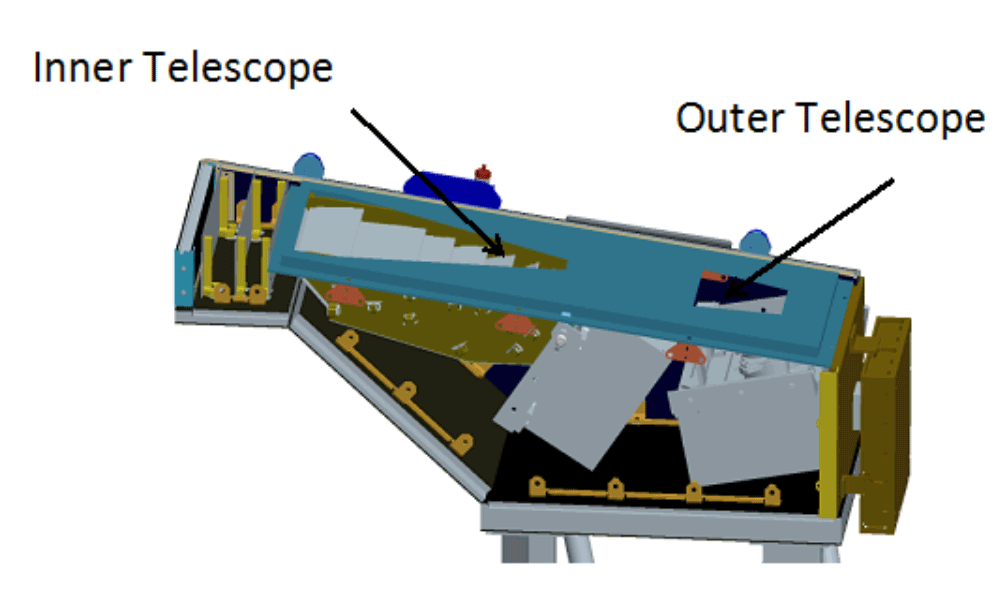
FIELDS : Cet ensemble d’instruments mesure les champs électromagnétiques, les émissions d’ondes radios et les ondes de plasma dans la couronne solaire. Il comporte cinq antennes qui mesurent la tension électrique et de trois magnétomètres. Quatre des cinq antennes sont placées à la base du bouclier thermique tandis que la dernière est pointée à l’opposé du Soleil. Les quatre antennes sont directement attaquées par le rayonnement solaire ce qui fait monter leur température à plus de 1 500K. Pour les protéger, la partie la plus longue de celles-ci est faite d’un tube de 2m en niobium C-103, matériau qui était utilisé pour les capsules Apollo et aujourd’hui dans les tuyères des moteurs Merlin de SpaceX. Cette première section est prolongée par une seconde de 30cm en molybdène, un métal dont la température de fusion est d’environ 2 900K. Cette dernière sert de bouclier thermique et électrique. La cinquième antenne, plus courte, est montée sur un mât déployable à l’arrière de la sonde. Les trois magnétomètres sont également fixés à ce mât long de 3,5m. Cette série d’instrument FIELDS a été développée par l’Université de Berkeley en Californie sous la direction du professeur Stuart Bale.
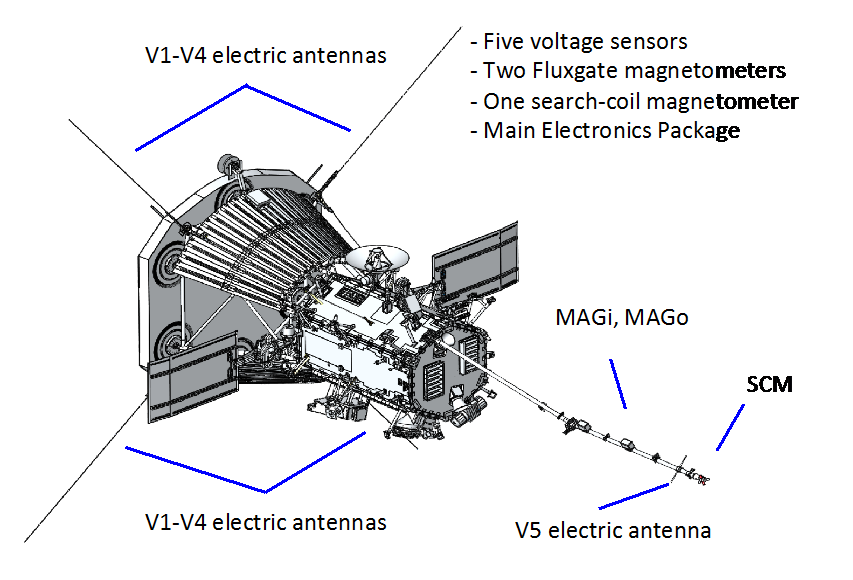
ISIS (Integrated Science Investigation of the Sun) will measure the characteristics of particles in the solar atmosphere that are accelerated to high energies (from 25 keV to 200 MeV). The eV (electron-Volt) is a unit of energy, equivalent to the energy of an electron in an electrical circuit with a voltage of one volt. Based on the data collected by ISIS, it will be possible to determine the origin of the particles and the conditions that led to their acceleration, as well as the role of particle collisions, magnetic field reconnections, and turbulence waves in the acceleration process. This instrument has two sensors: EPI-Lo and EPI-Hi. EPI-Lo measures the characteristics of solar wind ions with energies ranging from 25 to 1,000 keV. It consists of 80 small sensors, each equipped with a precise field of view, allowing for the mapping of an entire hemisphere. The second part of ISIS, EPI-Hi, measures more energetic ions, from 1 to 200 MeV, as well as electrons with energies ranging from 0.5 to 6 MeV. EPI-Hi consists of three telescopes which, together, provide the sensor with five wide fields of view. ISIS is positioned farthest at the back of the probe (opposite the Sun) and in the direction of motion. This arrangement gives the sensors a field of view of up to 10° (always with the direction of the Sun equivalent to 0°). This instrument was developed by Princeton University under the direction of Dr. David McComas.
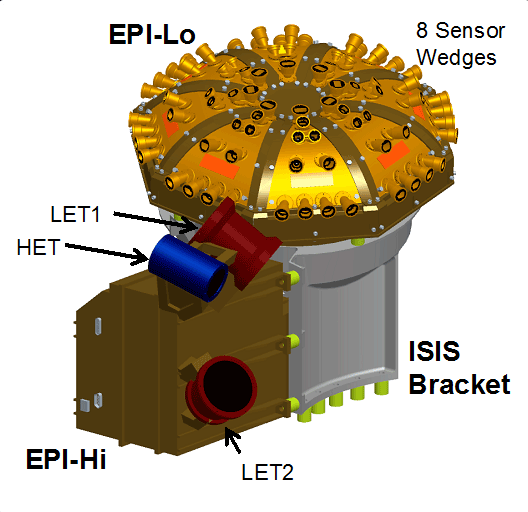
SWEAP, for Solar Wind Electrons Alpha and Protons, is a set of four instruments that will characterize electrons, protons, and alpha particles (helium nuclei): the main components of the solar wind. Through its data combined with those from other instruments, SWEAP will enhance our understanding of the heating and acceleration mechanisms at work in the solar corona. The first of the four SWEAP sensors is the SPC (Solar Probe Cup). This is a Faraday cup, an electron trap consisting of a conductive metal capsule that determines the number of intercepted electrons by measuring the produced current. Thus, the SPC will measure the flux and direction of arrival of ions (alpha particles and protons) and electrons. It is positioned at the edge of the thermal shield and facing the Sun, with a field of view of 60°. The other three sensors are part of the SPAN (Solar Probe Analyzers) family, which measures the arrival vector and velocity of electrons and ions with high temporal and energetic angular resolution. The two SWEAP SPAN A sensors perform these measurements in the direction of the probe’s movement, while sensor B performs them in the opposite direction. This set of sensors was developed by the University of Michigan and the Harvard-Smithsonian Center for Astrophysics under the direction of Professor Stuart Bale, who also led the fabrication of the FIELDS instruments.
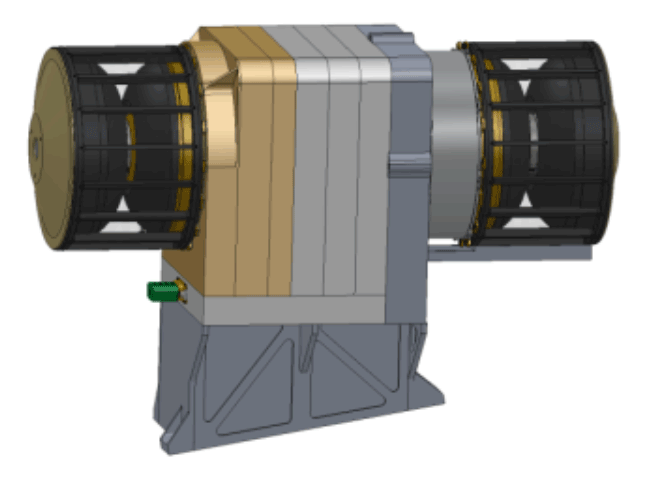
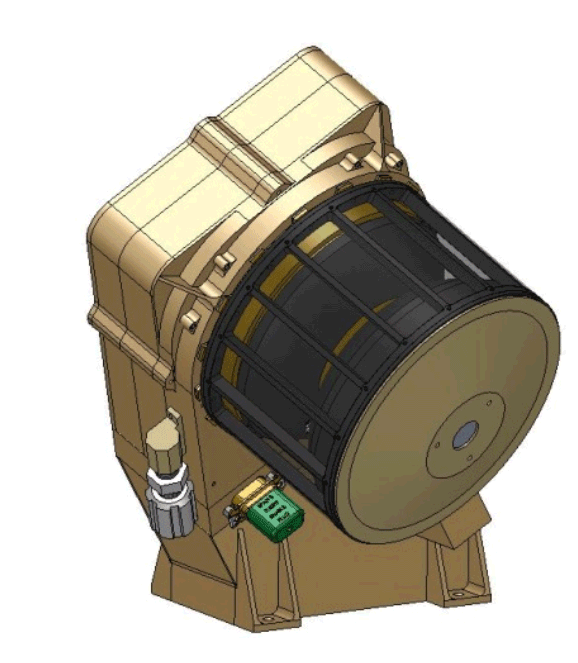
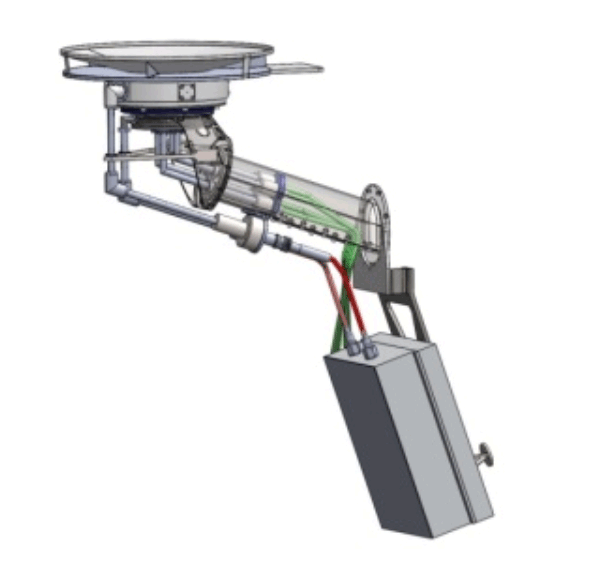
Technical Specifications
Parker Solar Probe is a compact spacecraft weighing only 685 kg, with 50 kg dedicated to scientific instruments. It is built around a hexagonally shaped prism platform with a one-meter diameter, housing a fuel tank at its center. Above this platform, a next-generation heat shield is attached by six beams. In total, the spacecraft measures 3 meters in height and 2.3 meters in diameter with all its equipment folded (antennas, mast, and solar panels).
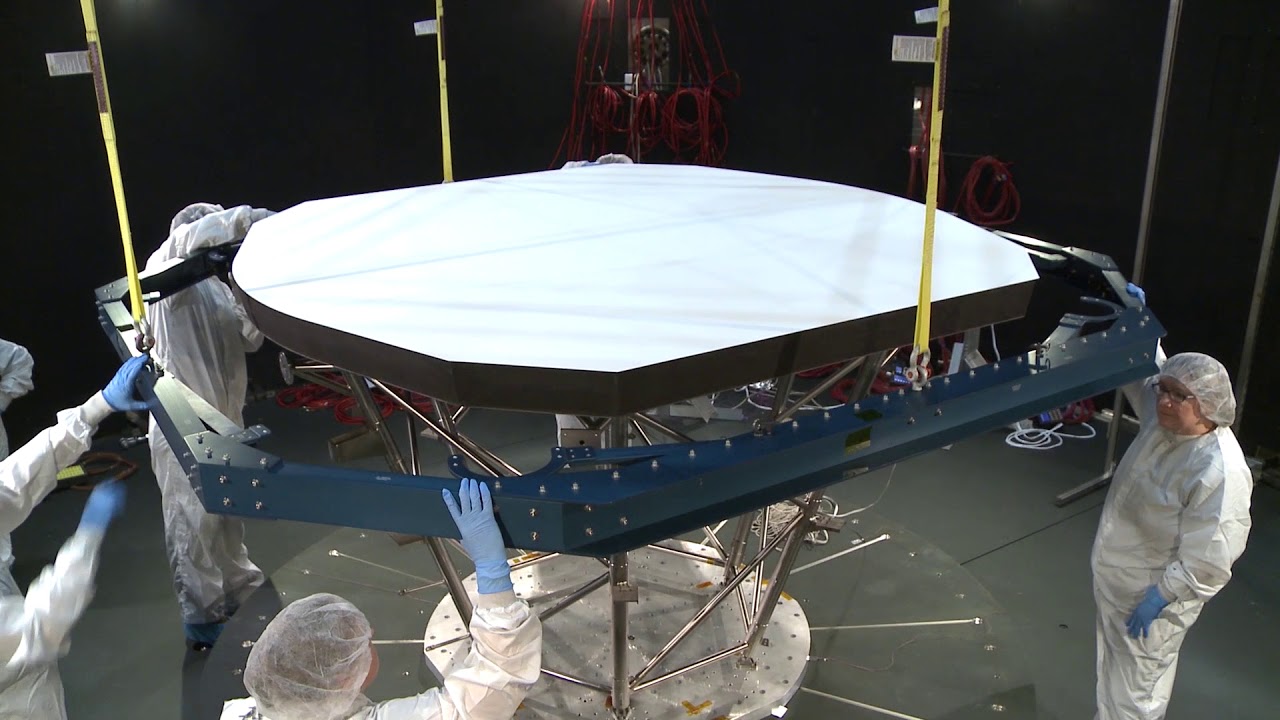
The thermal shield must intercept nearly all of the solar radiation flux, which is nearly 500 times more intense than that received in Earth orbit when the probe is closest to the Sun. This shield enables Parker Solar Probe’s instruments to maintain a temperature of 20K, while the side facing the Sun reaches up to 1,400K. The shield, which is 11.5cm thick and 2.3m in diameter, consists of carbon foam wrapped in a carbon-carbon composite (similar to that used on the leading edges of the wings of the American Space Shuttle) and coated with a layer of aluminum on the Sun-facing side. This shield has been cut to form two straight sides to allow for uniform exposure on the solar panels. The corners have also been trimmed to accommodate solar limb detectors, which we will discuss in a few lines.
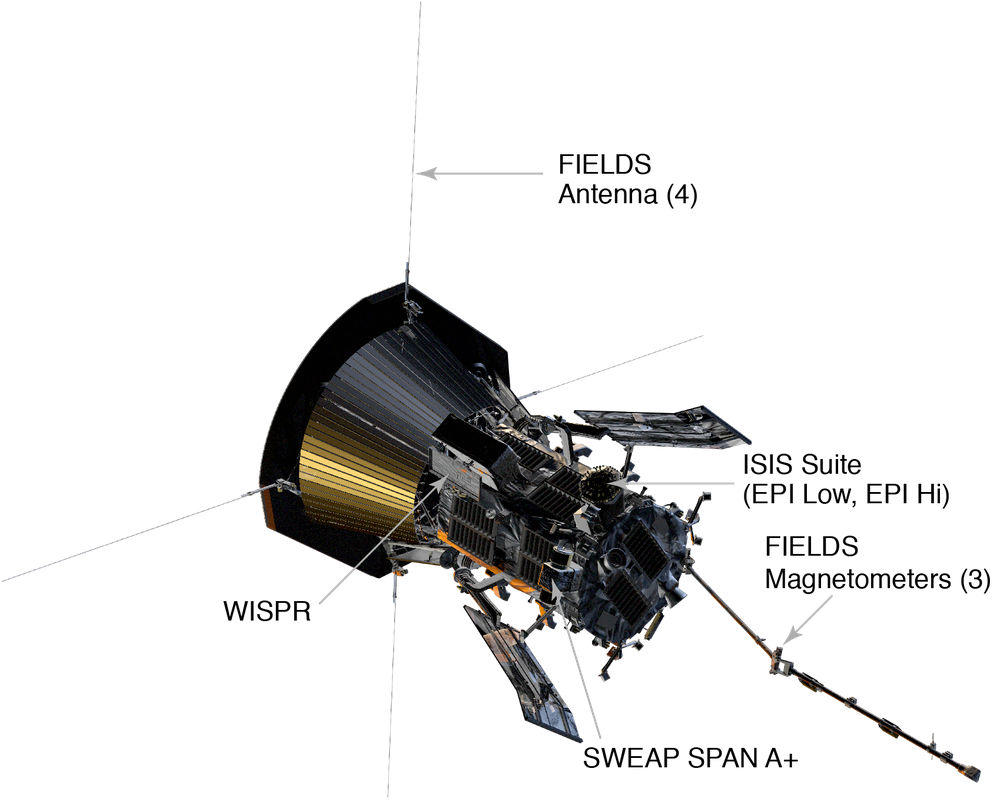
The spacecraft’s electricity is provided by a set of two photovoltaic panels. Each panel has an area of 0.65 square meters and must be able to function even at the closest approach to the Sun. To withstand extreme temperatures, they are folded within the shadow of the thermal shield. Each panel consists of a primary part (72 x 65 cm) completely in the shadow when the probe is at its perihelion, and a secondary part (27 x 65 cm) at its end, which is always exposed. To optimize the incidence of light radiation, the secondary panels form an angle of 10° with the primaries during passages at perihelion. To dissipate heat from these panels, a water cooling system passes through them and removes about 6,000 watts of heat to keep the solar cells below 160°C. This cooling system thus uses four radiators (with a total area of 4.4 square meters) arranged on the beams that hold the thermal shield to cool the water. The radiators consist of titanium tubes through which water flows and aluminum fins that dissipate heat. They are also used at aphelion to maintain sufficient heat within the probe. At its closest approach to the Sun, the panels produce 340 watts of electricity. Parker Solar Probe also has a 25Ah lithium-ion battery to store the generated energy.
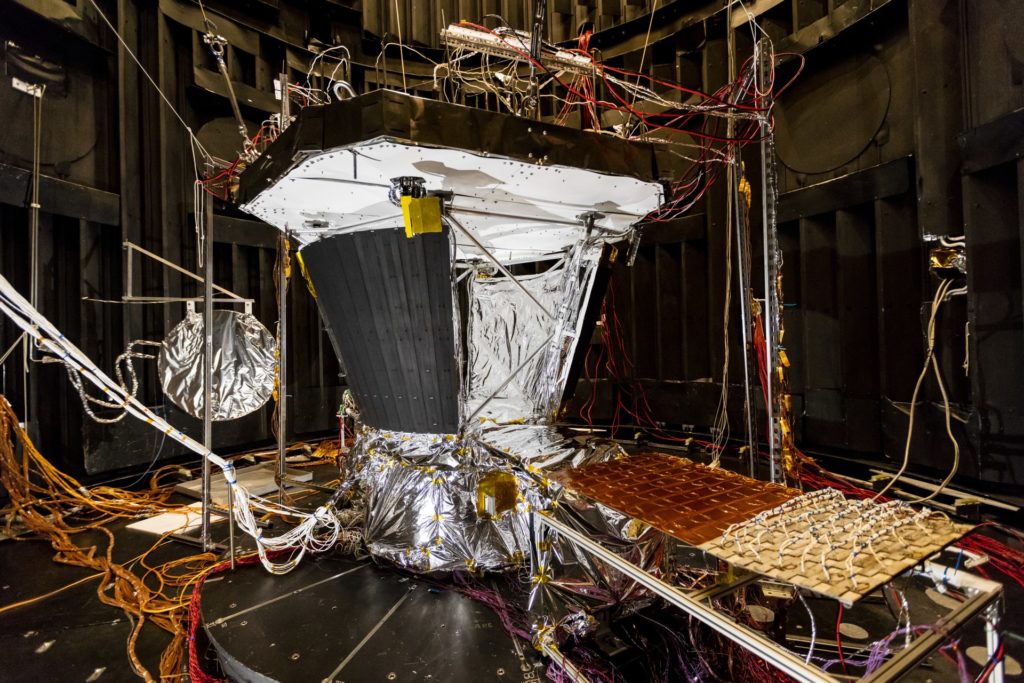
During such a mission, maintaining perfect attitude is crucial. Indeed, a 1° orientation error increases the energy to be dissipated by the probe by 35% at perihelion. To achieve this, Parker Solar Probe has a highly efficient attitude control system to keep its thermal shield constantly facing the Sun. The probe is stabilized on three axes using four reaction wheels and twelve small hydrazine thrusters. The internal tanks carry 55kg of propellant for a total velocity change of 170m/s over the entire mission duration. These thrusters are also used for trajectory corrections and for desaturating the reaction wheels. To determine its attitude, Parker Solar Probe relies on two star trackers mounted at the base of the platform as well as seven solar limb sensors. These sensors are positioned around the perimeter of the platform and at the end of the thermal shield’s shadow. It is these sensors that alert the onboard computers if the probe is no longer properly pointing its shield at the Sun. Additionally, there are two digital solar sensors that perform the same task as the previous seven, but when the probe is more than 0.7 AU from the Sun. Two inertial measurement units also provide all parameters related to rotation. The avionics (onboard computers) are designed so that the sensors and actuators remain operational even when the probe enters survival mode at perihelion. Thus, the control system has two redundant computers, each with three redundant processors.
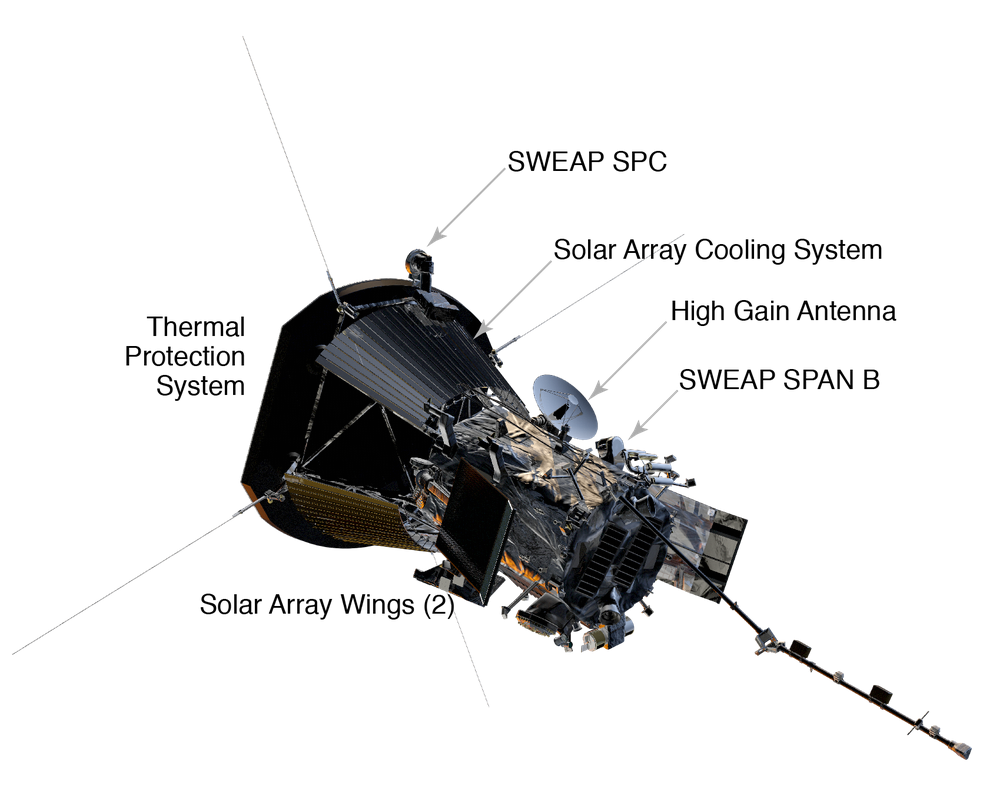
Finally, telecommunications are conducted in X-band and Ka-band using a high-gain antenna and several low-gain antennas. Scientific data transmissions occur only when the probe is more than 0.56 AU from the Sun. On the other hand, commands sent from Earth and telemetry data sent by the probe are continuously transmitted using two low-gain antennas. To store data during the periapsis passage, Parker Solar Probe has two redundant 32 GB storage spaces.
Déroulement de la mission
Parker Solar Probe was launched into orbit aboard a Delta IV Heavy rocket on August 12, 2018, at 9:31 CEST from Space Launch Complex 37 at Cape Canaveral. For this mission, engineers needed to send the probe very close to the Sun, requiring a very powerful launcher. According to Tory Bruno, CEO of ULA, only the Delta IV Heavy could perform this launch. Indeed, the characteristic energy of this mission is 154 km²/s², which is enormous. Simply put, the velocity Parker Solar Probe must reach when leaving Earth’s influence must be more than 12.4 km/s higher than Earth’s escape velocity (approximately 11 km/s). Initially, the probe was supposed to be launched aboard an Atlas V 551 equipped with a third stage using a solid-fuel Star 48GVX, which would have been developed specifically for this mission and would have had 50% more power than the usual Star 48B. This stage was tested in early 2014, but to reduce the risk of mission failure, it was decided to launch the probe with a Delta IV Heavy and a Star 48B stage that has already demonstrated its reliability on multiple occasions.
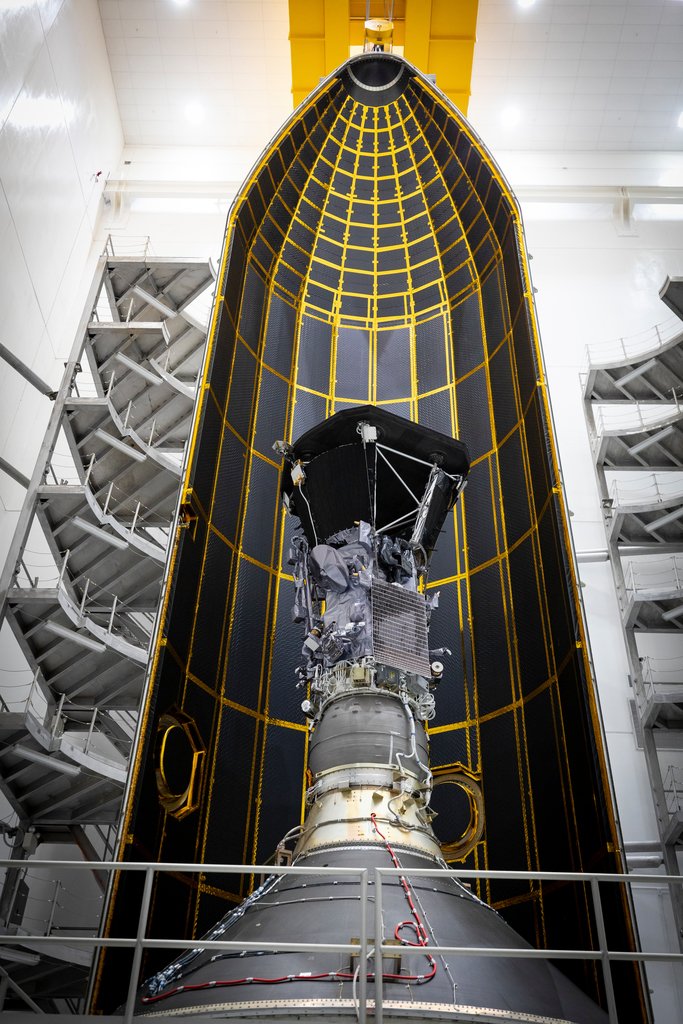
Six weeks after launch, the probe will reach Venus for the first time to take advantage of its gravity to further decrease its perihelion. Its first close pass by the Sun will occur at the end of 2018 at 0.163 AU (36 solar radii) from our star. Over the following years, the solar observatory will make six more close passes by Venus to progressively decrease both its perihelion (down to 0.044 AU, or 9.86 solar radii) and its aphelion, which will decrease from 1 AU to 0.8 AU. The orbital period will vary from 168 days for the initial orbits to 88 days for the later ones. An orbit is divided into two phases: the science phase, lasting about 11 days, which occurs when the probe is within 0.25 AU of the Sun, and the cruise phase, which lasts between 158 and 77 days. During the science phase, the solar panels are folded, communications are limited to commands and telemetry, data is stored, and no engine maneuvers are performed. Once out of this phase, the solar panels are redeployed, but it will wait until reaching 0.56 AU to deploy the high-gain parabolic antenna that will transmit instrument results in the Ka band. These results will be captured by the 34-meter diameter parabolic antennas of the Deep Space Network during 10-hour daily sessions. Venus flybys involve specific activities that begin 30 days before and end 10 days after the flyby. Among these activities are one or two trajectory correction maneuvers (TCMs) using the propulsion system.
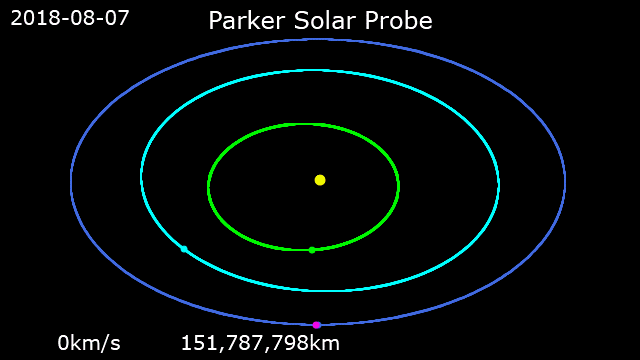